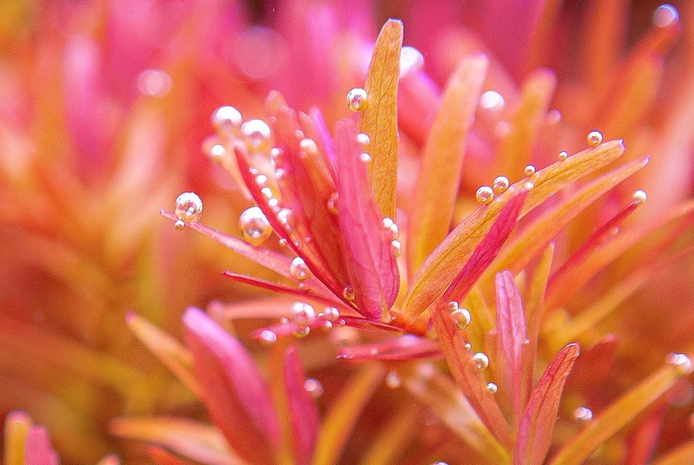
Aquatic plants need micronutrients to thrive and to grow just like all other living organisms.......
Boron
Boron (B) is one of the least understood micronutrients showing widespread deficiency in plants around the globe (Brown et al., 2002). B nutrition-mediated physiological and metabolic activities that reduce disease susceptibility in the plant system are attributed to (1) strengthening cell wall structure through the formation of carbohydrate-borate complexes, which control carbohydrate transport and cell wall protein metabolism, (2) controlling cell membrane permeability and stability function, and (3) phenolics or lignin metabolism (Brown et al., 2002). In B deficient conditions, plant cell walls tend to swell and split, resulting in weakened intercellular space, which eventually weakens the physical barrier to the initial infection (Blevins and Lukaszewski, 1998). Sanjeev and Eswaran (2008) observed that B nutrition contributed to the maximum fungal mycelial growth inhibition and it can be used as a prokaryotic inhibitor at a certain concentration. The response of soil-borne phytopathogenic prokaryotes, such as Ralstonia, Pectobacterium, and Pantoea, to B can be assessed, and if boron concentration is not toxic to other beneficial plant-associated microorganisms, then altered B nutrition can be used as disease management effective means against the soil-borne plant pathogens.
Zinc
Zinc (Zn), one of the crucial micronutrients, plays its role in plant response toward phytopathogens primarily activating or stabilizing metalloenzymes (Fones and Preston, 2012). Generally, the Zn deficient plants are more prone to pathogen attack (Streeter et al., 2001), thereby providing Zn the status of a significant element deciding the outcome of the plant-pathogen interaction. This results in limiting the invader’s entry or evading plant defense responses. Several studies suggest Zn fertilization role in reducing plant symptoms (Li et al., 2016; Machado et al., 2018); however, an increased susceptibility toward other pathogens was also reported due to protective Zn concentrations used against certain pathogens of the same host (Helfenstein et al., 2015). The studies conducted on the potential relationship between Zn availability status and fungal disease severity have reported an alleviated disease response in plants supplemented with Zn (Huber and Haneklaus, 2007; Khoshgoftarmanesh et al., 2010) while the contrary results were observed in soybean plants with varied Zn treatment, wherein either normal or high Zn fertilization had fewer positive counts for bacterial pustules caused by Xanthomonas axonopodis pv. glycines (Helfenstein et al., 2015). An evolutionary-conserved Zn-sensing phenomenon connecting root growth to pathogen response mechanism was stated by Bouain et al. (2018). In this study, the authors found that azelaic acid triggered by Azelaic Acid Induced1 (AZI1), belonging to the lipid transfer protein family (LTP) of the pathogenesis-related (PR) protein during systemic acquired resistance (SAR), regulated the plant growth and immunity responses on the basis of Zn availability status in plants.
Copper
Copper (Cu) is one of the significant micronutrients required by plants that acts as a cofactor for several enzymes involved in respiration and electron transport proteins (Sommer, 1931). Cu is a plant protection essential part of controlling oomycetes, fungi, and bacteria for over a century. Although diseases can be managed by Cu applications, the lack of curative or systemic activity leads to Cu spray applications year after year (Graham et al., 2011). Plants with low Cu content show an increased disease incidence as a result of reduced lignification (Marschner, 1995). Cu fertilization in plants reduces the severity of fungal and bacterial diseases associated with cell wall stability and lignification (Broadley et al., 2012). The best evidence of a Cu effect on host plant resistance to disease can be observed in cases where Cu is applied in soil, and it lowers the leaf infection as evident in powdery mildew in wheat and ergot (Claviceps sp.) (Evans et al., 2007). The Cu synergistic effects can also be stated when it was used with other fungicides, such as Mancozeb, which leads to a reduction in canker and fruit spotting symptoms (Shoemaker, 1992). Cu compounds and their different combinations, in different studies, are reported to reduce sheath blight severity (Rhizoctonia solani) in rice (Khaing et al., 2014) and bacterial canker (Clavibacter michiganensis subsp. michiganensis) in tomatoes (Bastas, 2014).
Manganese
Manganese (Mn) is an important micronutrient known for its efficacy on pathogen and resistance development in plants (Huber and Graham, 1999) owing to its ability for phenolic and lignin compound synthesis (Broadley et al., 2012). Cacique et al. (2012) reported that high Mn concentration on leaf tissues was found to decrease blast symptoms by Pyricularia oryzae in rice. Heine et al. (2011) observed that Mn can also contribute to black leaf mold disease control (Pseudocercospora fuligena) in tomatoes. Plants with inadequate Mn nutrition are observed to be unable in restricting the fungal hyphae penetration into the root tissues (Graham and Webb, 1991) while plants with adequate Mn nutrition show an alleviation in lignification and a reduction in aminopeptidase and pectin methyl esterase synthesis that is required essentially for fungal growth and for host cell wall breakdown, respectively (Carrow et al., 2001).
Iron
Iron (Fe) is an essential micronutrient required by plants and pathogens having both positive and negative effects on the host and host disease resistance (Kieu et al., 2012; Aznar et al., 2015). Fe catalyzes ROS production that is used by the plant for alleviating the local oxidative stress as a defense response against the pathogens, thereby making iron play an intricate role in plant-pathogen interaction (Aznar et al., 2015). Fe fertilization is evident to be effective in antimicrobial compound synthesis leading to an indirect effect on the plant’s metabolic activity (Aznar et al., 2015). A reduction in symptom severity and pectate lyase encoding gene expression of the two soft rot-causing pathogens, such as Dickeya dadantii and Botrytis cinerea, was observed in plants showing Fe starvation (Kieu et al., 2012). Fe is also reported to enhance the fungal growth in certain plant-fungus interactions, as it was observed in Phytophthora parasitica var. nicotianae, wherein the fungal growth was observed to enhance in Fe3+ supplemented synthetic glucose asparagine medium (Hendrix et al., 1969). Fe also plays a potent role in Pseudomonads that are adapted to produce iron-chelating agents called siderophores in Fe-deficient soils, which, in turn, suppress certain fungal pathogens by starving them of iron (Calvent et al., 2001). Siderophores are also involved in some volatile antibiotic compounds’ synthesis (Thomashow, 1996). Depending on the host, the defense activation mechanism involves either their Fe scavenging property or receptor-mediated recognition as in the case of pattern-triggered immunity (Aznar and Dellagi, 2015). The reduced iron availability for fusaria-related wilts induced by fluorescent pseudomonads producing siderophores is reported as the main mechanism to reduce disease incidence in fusarium wilt of tomatoes (De Weger et al., 1986; Alabouvette, 1999; Hussain et al., 2016). In fact, soil suppressiveness to fusarium wilt of tomatoes has been mainly ascribed to Fe competition between the pathogenic Fusarium oxysporum isolates from the rhizosphere with the wild populations of fluorescent pseudomonads (Haas and Défago, 2005; Lemanceau and Alabouvette, 2008).
Silicon
Silicon (Si) is not essentially a micronutrient but stands out eminently in its potential for decreasing several pathogens’ severity in varied crops belonging to the families Poaceae, Equisetaceae, and Cyperaceae (Huber et al., 2012; Pozza et al., 2015). The increased Si supply strongly reduces the number of lesions on young leaves, indicating an increase in disease resistance (Osuna-Canizalez et al., 1991). The silicates are known for inducing defense responses in plants by involving cell wall strengthening through alleviated phytoalexin production, increased lignification, PR protein synthesis, and phenolics production (Fawe et al., 2001; Oliveira et al., 2012).
Silicon is accumulated mainly in epidermal cells and exclusively on endodermal cells in roots and creates a physical barrier for fungal hyphae penetration into plant roots (Najihah et al., 2015). An increase in the activity of antioxidative enzymes (peroxidase, polyphenol oxidase, phenylalanine ammonia lyase, and lipoxygenase) was also observed after Si application (Shetty et al., 2011), which are considered the second line of defense for the pathogen entry into the host (Pozza et al., 2015). A significant reduction in lesion length of bacterial leaf blight (Xanthomonas oryzae pv. oryzae) among four rice cultivars was reported by Chang et al. (2002) following Si application, which was correlated with the soluble sugar content reduction in plant leaves amended with Si. Reduced severity in rice sheath blight disease was attributed to the increased lignin content and enhanced activities of antioxidative enzymes in rice leaves with Si addition. Therefore, knowing its effects on disease reduction, it can be included as an important component of crop protection.
Conclusion
Since sustainable agriculture that uses increasing amounts of bio-fertilizers and organic amendments from a wide range of organic wastes represents a very important plant mineral nutrient source, it is fundamental to know the mechanisms of action by which such minerals can be involved in plant defense in several pathosystems. It is a general assumption that balanced nutrition leads to a healthy plant, which reduces disease susceptibility and infection. Thus, it is important to provide balanced nutrition at the due time when the nutrient can be most effectively used for disease control. Nutrient manipulation achieved by either modifying the nutrient availability or modifying the nutrient uptake for disease management or suppression has been reported in several studies. Fertilizers’ application affects plant disease development under field conditions either directly through the plant’s nutritional status or indirectly by affecting the conditions, which can influence the disease development, such as dense stands, changes in light interception, and humidity within the crop stand. It is a general assumption that balanced nutrition leads to a healthy plant, which reduces disease susceptibility and infection. Thus, it is important to provide balanced nutrition at the due time when the nutrient can be most effectively used for disease control.
Add comment
Comments